By Vidya Rajan, Columnist, The Times
Eyes harvest light reflected from objects. Optic nerves transmit that information to the brain. The brain turns that information into an image. Thus eyes are the intermediary between an object and its perception. Let’s look at them more carefully. Don’t shrink from anatomical detail or biochemistry – this is fun stuff. It’s fun because delving into biomechanical intricacies is both stimulating and informative.
Studies on animal eye evolution shows that eyes originated as light-sensitive patches on the skin, then progressed through depression “eye cups” to chambers containing a lens on one end and a light-sensitive retina on the other. A huge diversity of living animals demonstrating various eye manifestations exist [1]. [A short video at https://youtu.be/duBW9QabXfw summarizes the hypothetical development of light sensing patches to eyes.] The retina contains a variety of cells with pigments that absorb different wavelengths of light. These pigments have two components – a functional protein, opsin, and its lipid cofactor, retinal. (Cofactors are substances that help proteins to perform their function – ex. iron is a cofactor of hemoglobin.) As discussed in an earlier article [2], 11-cis retinal isomerizes to all-trans retinal when it absorbs energy from light. Retinal is a common responding factor, but the protein opsin fine-tunes that response: rods work in dim light and cones are wavelength-specific and vary between animals. In humans, rods contain rhodopsin and cones contain three types of photopsins that absorb at different portions of the spectrum as seen in Figure 1 below: S responds to blue light wavelengths, M to green light wavelengths and L to red light wavelengths. Rhodopsin in rods absorbs best in the blue-green region but has a broad ability to be triggered by wavelengths across the visible spectrum.
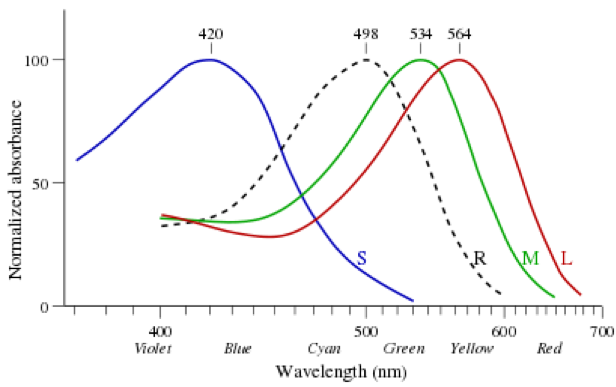
Figure 1: Normalized absorption spectra of the three human photopsins and of human rhodopsin (dashed line). Reproduced under Creative Commons License. Vectorized version of the GFDL image Cone-response.png uploaded by User:Maxim Razin based on work by w:User:DrBob and w:User:Zeimusu. – After Bowmaker J.K. ; and Dartnall H.J.A., “Visual pigments of rods and cones in a human retina.” J. ; Physiol. 298: pp501-511 (1980).
Opsin proteins are encoded in DNA in the genome and function as gates for positively-charged sodium ions: gates whose opening and closing is controlled by cofactor 11-cis retinal/all trans retinal isomerization. 11-cis retinal-linked opsin gates are open allowing a stream of ions to cross from one side of the retinal membrane to the other. This sets up a current, called “dark current” by the boffins, making the inside of the cells a little less negative. When light falls on the retinal cell, the channel closes. Now that sodium cannot enter the cell, the cell becomes rather more negative and the voltage difference between the inside and outside, called the potential difference, increases. Like a dam when its sluice gates are closed, pressure builds up until boom! a nervous impulse is triggered. In a series of events we don’t need to sweat here, the optic nerve carries these images to the visual cortex where they are perceived as an image.
It is said “beauty lies in the eyes of the beholder”. The question is, do all beholders see the same image? Beekeepers regularly go to talks where they are shown pictures of what “bees really see” – life in the ultraviolet zone: flowers that have hues we don’t see, with “landing strips” that guide them to the nectary (see Figure 2) with enhanced contrast between light and dark bands. Much is also made of their ability to detect polarized light and thus the location of the sun, even on a cloudy day. This is worth breaking down: 1. How do bees see color? and, 2. How do bees sense polarized light?
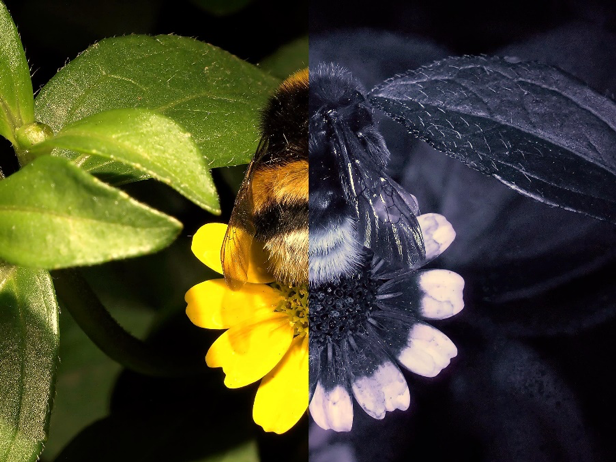
Figure 2: Bee-vision in the ultraviolet range. Mapping the colors of a bumblebee sitting on a flower as seen in human (left) and bee vision (right). Note that this image only examines the UV spectrum. Bees also have the ability to see yellow, so the outer, white portions of the petals will appear yellow. In addition, bees produce multiple images of the object due to the presence of ommatidia, thus they see a more elongated, multiple and blurry image. (C) Dr Schmitt, Weinheim Germany, uvir.eu
For an interactive bee vision simulation, see http://andygiger.com/science/beye/beyehome.html
First, let’s examine the process of seeing in color in humans and compare it to color vision in bees. When sunlight falls on an object such as your red shirt, the red dye in your shirt absorbs all the other visible light wavelengths, reflecting only the red wavelength. Thus another human who has L photopsins sees you wearing a red-colored shirt. Bees lack L photopsin and cannot see red, but they have instead a photopsin in the UV wavelength making UV reflecting regions seem bright (see Figure 2). What color will your shirt appear? It’s hard to imagine what not being able to see red might “look” like, until you realize that UV is invisible to us humans. Red is invisible to bees. We don’t see UV – they don’t see red. Maybe they see grey, like someone with colorblindness; I don’t know if we even know. But they don’t see a red shirt, that’s the bottomline.
If bees can’t see red, how come there are so many red flowers in nature? For one thing, bees probably see them reflecting ultraviolet, but largely it’s because birds and mammals also provide pollination services to specific partners. Bats pollinate the long, tubular flowers of tropical vines, and spread the seeds of plants like figs by eating the fruit and defecating seeds embedded in a little packet of fertilizer. Birds also are renowned pollinators. Who has not thrilled to see a little hummingbird dip its long beak into a red cardinal flower? Birds have L, M, S and UV photoreceptors. If birds appear colorful to us, think how much more spectacular they must appear to each other!
Now to polarization. When light emanates from a point source it is unpolarized, with waves propagating in every which direction. Polarization is a way to detect waves in just one plane, as a way to clean up “haze” around light emanating from a point source (see Figure 3). A circular polarizing filter does the same except that the polarizing filter can select either clockwise or counterclockwise polarized light. A trick that optics can do is to overlap two images with different polarizations into, say, a moving image, and then give you glasses with two lenses with different polarizations to look through at the movie. This produces two separate images in each eye, which your brain reads as a single overlapping image, and suddenly you’re watching a 3D movie! Well, bees watch such 3D movies all the time using the ability to polarize light, but without the need to wear a clunky pair of paper glasses. Polarization removes reflective haze and provides clarity to the image (see Figure 3, right).
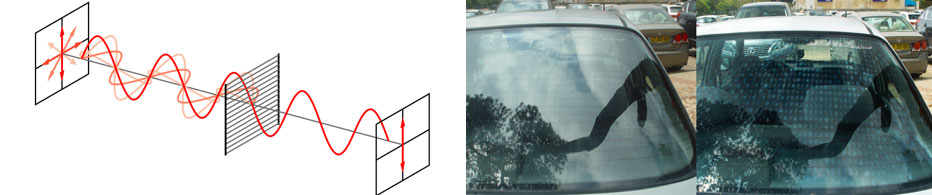
Figure 3: Left: Mechanism of polarization of light. Right: Comparison of unpolarized (left) and polarized (right) lens. From: Left: https://commons.wikimedia.org/wiki/File:Wire-grid-polarizer.svg#file Right: Etan J. Tai, https://en.wikipedia.org/wiki/Sunglasses#/media/File:CarWindowPolarization.jpg. Reproduced under Creative Commons Attribution-Share Alike 3.0 Unported license.
The most acute vision on Earth belongs, hands down, to the mantis shrimp. This “humble” bottom feeder rocks 16 photopsins and the kind of vision that we may encounter in a kaleidoscope during a fireworks show. In addition, the mantis shrimp has three independent regions in each eye with trinocular independently operating in each eye, allowing each to swivel autonomously and operate independently. Although its clothing color palette makes it look like it dressed in the dark, the mantis shrimp can distinguish not only colors that we can see in many more shades, it can see above and below the “visible” spectrum in both ultraviolet and infrared.
What we don’t yet know is whether they dream in color, or in black and white.
References:
- Genetic Science Learning Center “Eye Evolution”. Learn. Genetics. 2013 [cited 2018 August 27]; Available from: https://learn.genetics.utah.edu/content/selection/eye/.
- Rajan, V., Aposematism: Worn Warning, in The Unionville Times. 2018.
URL: https://www.unionvilletimes.com/?p=40003